I'll give you an example calculation for a wavelength of 532 nm. At refractiveindex.info, we find that the complex refractive index of aluminum at that wavelength is $n_1 = 0.938777 + 6.4195i$. Let's also assume that the light is incident normal to the surface, from air ($n_0=1$), and the aluminum layer is on a glass substrate ($n_2 = 1.5$).
(I'm naming the materials 0, 1, and 2 as in the picture below:)
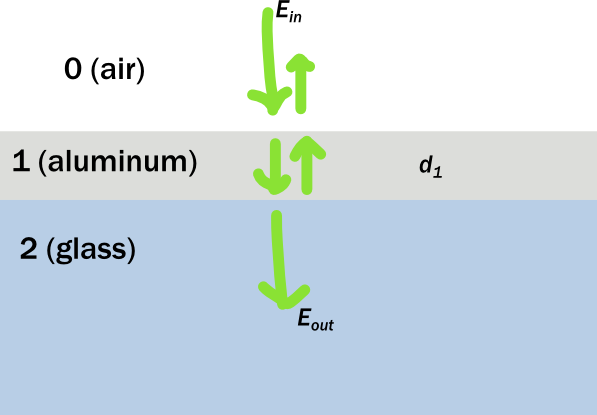
At each interface, you have the Fresnel amplitude reflection coefficient:
$r_{jk} = \frac{n_j - n_k}{n_j + n_k}$
Note that this formula is simplified for normal incidence. Note also that $r_{kj} = -r_{jk}$ and the transmission coefficient $t_{jk} = 1 + r_{jk}$ (for perpendicular polarization only though... but doing the calculation for parallel polarization is much the same.)
During propagation through the aluminum, the field undergoes a phase delay and attenuation (which I will combine into the complex constant $\delta$, equal to $e^{ik_1d_1}$, where $d_1$ is the thickness of the aluminum layer and $k_1$ is the wave number in the aluminum layer, $k_1 = 2\pi n_1 / \lambda$.
So, to calculate the transmission $T=|E_\text{out}|^2/|E_\text{in}|^2$ we have several contributions that we need to sum, while preserving their phase. We have a contribution from the direct transmission,
$t_{01} \delta t_{12}$,
a contribution that is reflected back and forth through the aluminum once,
$t_{01} \delta r_{12} \delta r_{10} \delta t_{12}$,
a contribution that goes back and forth twice,
$t_{01} \delta r_{12} \delta r_{10} \delta r_{12} \delta r_{10} \delta t_{12}$,
and so on.
The sum of these contributions is
$\frac{E_\text{out}}{E_\text{in}} = t_{01} t_{12} \delta \sum_{k=0}^\infty (r_{12} \delta r_{10} \delta)^k = (1+r_{01})(1+r_{12})\delta \sum_{k=0}^\infty (-r_{01}r_{12}\delta^2)^k$,
and this is a geometric series, so it becomes
$\frac{E_\text{out}}{E_\text{in}} = \frac{(1+r_{01})(1+r_{12})\delta}{1 + r_{01}r_{12}\delta^2}$,
so
$T=\left|\frac{(1+r_{01})(1+r_{12})e^{ik_1d_1}}{1 + r_{01}r_{12}e^{2ik_1d_1}}\right|^2$.
Note that you may have to multiply $T$ by a factor of $n_2/n_0$, I'm doing this from memory and I can never remember whether you have to do that or not. (I hope I didn't make any other mistakes... ;-)
Here's a plot that I calculated for 532 nm:
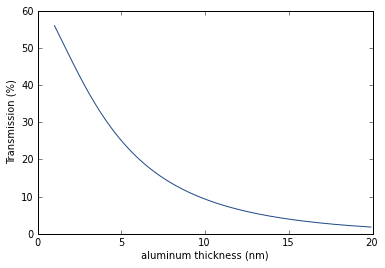
So this 40% occurs at around 3 nm thickness. You will be hard pressed to get an aluminum film of that thickness, as John Rennie points out. You will also find, as I have found to my detriment, that the optical properties of thin film aluminum are quite different from those of bulk aluminum, on which this calculation is based. Here are a couple references: (1) (2). The second one is open access. I'll pick a choice quote from the introduction of the first one:
It is well known that the optical properties of ultrathin metal films differ from those of bulk metal. This was most dramatically observed for the Al film.
So, picking an aluminum film with 40% transmission is not as easy as you might think.